Winning Strategy for Sweepstakes Participating in sweepstakes can be an exhilarating experience, offering the chance to win fantastic prizes. However, to increase your odds, it’s essential to implement a winning strategy. This article will explore effective tips and techniques that can significantly enhance your chances of winning sweepstakes. Understanding Sweepstakes Before diving into strategies, it’s crucial to understand what sweepstakes…
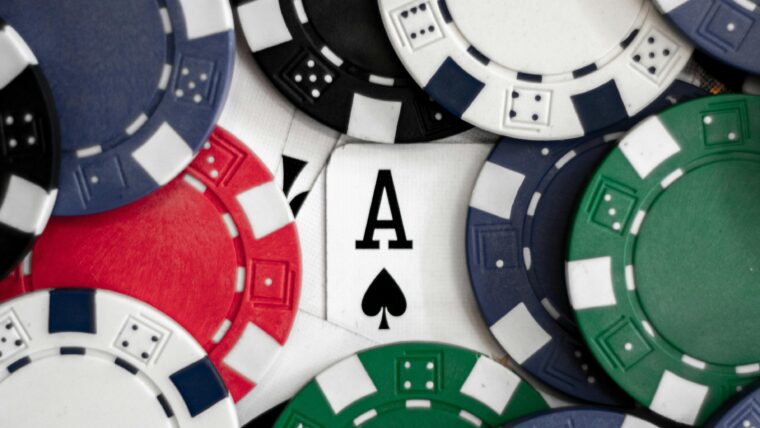